Marburg Virus Disease: Another Filovirus Zoonosis
Recently, Marburg Virus Disease (MVD), Ebola’s equally deadly cousin, emerged in Tanzania and in at least three separate locations in Equatorial Guinea. The two countries are on opposite sides of Africa’s coast and separated by thousands of kilometers, but both are in the range of Egyptian fruit bats. The bats are the natural hosts for both viruses that cause MVD, Marburg virus (MARV) and its close relative Ravn virus (RAVV) (~90% nucleotide sequence identity)(1). It is likely that these bats are a zoonotic source of MVD, either directly by human exposure to bat feces, blood or bites, or, less likely, indirectly transmitted through insect bites of bats and then people. It is not certain whether the bats represent the ultimate reservoir or instead acquire the marburgviruses from another species yet to be identified. Transmission also occurs from person to person and from exposure to bodily fluids from infected animals such as monkeys. But at this time, it is not clear whether there are other links that could explain the near-simultaneous geographically separated outbreaks.
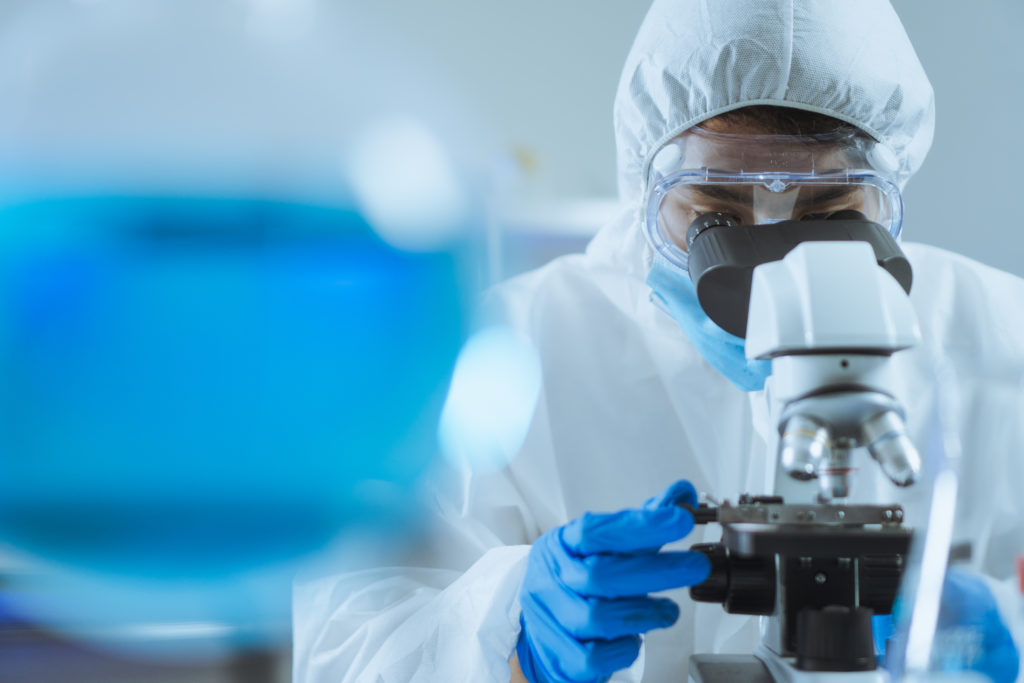
MARV was first identified in 1967 when German and Yugoslav lab workers became infected after working with tissues from infected grivet monkeys(2); 7 of 31 infected people died. MARV is one of three genera of filovirus, a group that includes Ebolavirus and Cuevavirus(3). MARV, similar to other filoviruses, has a single negative RNA strand genome of 19 kilobases that encodes 7 proteins, including a spike protein, GP.. The spike protein binds to the cholesterol transporter protein NPC1 to enable viral entry. NPC1 appears to be essential for infection both by MARV and by Ebola(4, 5) and in its absence cells cannot be infected.
The most concerning element of the recent outbreaks is that they have occurred closely spaced in time in different geographically dispersed regions, as aforementioned. In the absence of genetic analyses, it is not clear whether they are directly linked or are independent events, pointing out a serious need for better access to DNA-sequencing facilities in the areas affected. Previously, MARV closely related to those from an outbreak in Angola in 2005 was isolated from Egyptian fruit bats in West Africa(6). Similarly, Angola-like MARVs also have been recently isolated in Equatorial Guinea from Egyptian fruit bats closely related to (but not identical with) those from an infected patient in Guinea who died in 2021, which strongly suggests his infection was locally acquired(7). Infection in bats is not highly pathogenic and features an unusual muted inflammatory state(8).
Mitigation efforts in outbreaks have generally been restricted to classical isolation and supportive care. These are eventually effective, but take a toll on health care workers. There are no antivirals in clinical use for MARV, and there are no approved vaccines. Remdesivir has been shown to potently inhibit MARV and RAVV replication in vitro(9), but whether it will be clinically useful is unclear. More hopefully, remdesivir and neutralizing mAbs were strongly protective in a rhesus macaque model, either when given individually up to 5 days post-inoculation or in combination at 6 days after inoculation(10).
There are some experimental vaccines of limited availability (several thousands of doses) that may be sufficient in number for ring or targeted vaccination campaigns. But it is not feasible to conduct phase 3 vaccine trials for MARV because infections are not sufficiently widespread(11). So vaccine testing has been confined to animal models, of which non-human primates are by far the most relevant. Of the several MARV vaccines in development, one of these uses a recombinant vesicular stomatitis virus (rVSV) containing the Marburg GP and protected 80% against challenge in a cynomolgus monkey model with the Angola strain when administered 5 days before infection, although this protection fell to only 20% when given 3 days prior to challenge(12). Another study with cynomolgus monkeys using a similar vaccine showed that vaccination 7 days prior to challenge resulted in sterilizing immunity, neutralizing antibodies, and cellular immunity(13), suggesting that early treatment of humans will be superior to late. Other vaccine candidates, including adenoviral-vectored vaccines, inactivated viral vaccines, and virus-like particles, have been tested with varying degrees of efficacy (14), but the VSV-based vaccines seem the best developed.
One of the obvious difficulties in research involving MVD is its lethality; it requires BL4 facilities and is considered a Category A bioterrorism agent. It would therefore be highly useful to have a safe proxy for MVD to use in vaccine and other studies. One such construct has recently been described in which an essential gene was removed from MARV and transduced into a stable cell line(15). This provides the essential gene in trans, allowing the virus to replicate in the cell line but not in other cells, rendering it safe for in vitro studies, including those on neutralizing antibodies. The appearance of MVD in four geographically separated regions gives cause for concern, especially in the absence of information as to how they are linked. We clearly need effective vaccines, better local DNA sequencing infrastructure, effective antivirals, and a better understanding about the process by which MVD zoonoses occur. It’s not a virus to be taken lightly, and, as in the case of other zoonotic viruses, surveillance programs must be deployed as soon as possible if we are to avoid further loss of human life.
References:
- J. H. Kuhn, Guide to the Correct Use of Filoviral Nomenclature. Curr Top Microbiol Immunol 411, 447-460 (2017).
- W. Slenczka, H. D. Klenk, Forty years of marburg virus. J Infect Dis 196 Suppl 2, S131-135 (2007).
- M. Kerper, Y. Puckett, in StatPearls. (Treasure Island (FL), 2023).
- J. E. Carette et al., Ebola virus entry requires the cholesterol transporter Niemann-Pick C1. Nature 477, 340-343 (2011).
- M. Cote et al., Small molecule inhibitors reveal Niemann-Pick C1 is essential for Ebola virus infection. Nature 477, 344-348 (2011).
- B. R. Amman et al., Isolation of Angola-like Marburg virus from Egyptian rousette bats from West Africa. Nat Commun 11, 510 (2020).
- M. T. Makenov et al., Marburg virus in Egyptian Rousettus bats in Guinea: Investigation of Marburg virus outbreak origin in 2021. PLoS Negl Trop Dis 17, e0011279 (2023).
- A. D. Jayaprakash et al., Marburg and Ebola Virus Infections Elicit a Complex, Muted Inflammatory State in Bats. Viruses 15, (2023).
- S. R. Radoshitzky et al., Expanded profiling of Remdesivir as a broad-spectrum antiviral and low potential for interaction with other medications in vitro. Sci Rep 13, 3131 (2023).
- R. W. Cross et al., Combination therapy protects macaques against advanced Marburg virus disease. Nat Commun 12, 1891 (2021).
- Y. Q. George, J. Thibaut, W. J. Edmunds, Assessing the feasibility of Phase 3 vaccine trials against Marburg Virus Disease: a modelling study. medRxiv, 2023.2002.2022.23286294 (2023).
- W. Courtney et al., A highly attenuated Vesiculovax vaccine rapidly protects nonhuman primates against lethal Marburg virus challenge. bioRxiv, 2022.2001.2022.477345 (2022).
- K. L. O’Donnell et al., Rapid protection of nonhuman primates against Marburg virus disease using a single low-dose VSV-based vaccine. EBioMedicine 89, 104463 (2023).
- J. J. Suschak, C. S. Schmaljohn, Vaccines against Ebola virus and Marburg virus: recent advances and promising candidates. Hum Vaccin Immunother 15, 2359-2377 (2019).
- V. Bert et al., Development and optimization of biologically contained Marburg virus for high-throughput antiviral screening. bioRxiv, 2022.2007.2029.502006 (2022).