Further Thoughts on Viral Persistence
August 2, 2022
INTRODUCTION
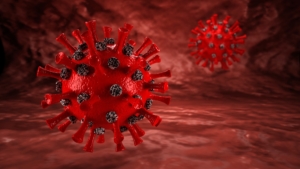
Our previous Perspectives focused on the question of whether persistent cryptic expression of virus might be contributory to long COVID. To further consider this question, it may be useful to look at examples of persistence by other viruses.
DNA VIRUSES
The clearest and most easily understood examples of persistence are found with the DNA viruses. Their genomes are naturally more stable than those of RNA viruses. Retroviruses such as HTLV-I and HIV are RNA viruses that produces DNA proviral form that can integrate in the host DNA. Herpesviral genomes persist as episomal DNA and the few viral genes expressed during latency ensure viral genomic duplication upon cell division. Human papilloma virus establishes its genome in the cell nucleus and integration of a partial genome into the host cell genome can cause cervical, penile, anal, and oral cancers. Another example is the adenoviruses, which can reemerge following immune suppression
RNA
It’s less clear how RNA viruses can persist as RNA is not intrinsically very stable. One obvious way is for infection to be not completely cleared, which we considered in our previous Perspective. An alternative theme in RNA viral persistence is the occurrence of defective viral genomes, generated by partial deletions or partially incapacitating mutations, generating a non-replicative virus but one that can still express viral proteins. If the viral replicative genes are preserved and expressed, persistence of the defective genome would be possible. The deletions can be generated by the replicase falling off the viral RNA template, then reattaching elsewhere. If this occurs in a cell infected with two different strains of the virus, the result can be a recombinant virus. Alternatively, defective viruses can be generated by mutations caused by error prone replicases or host-encoded RNA editing enzymes. A good review on this process is found in . It should be emphasized that persistence by these defective viral genomes (DVGs) is distinct from the presence of replicating virus in cryptic sites; we will focus here on DVGs rather than persistent undetected replication, which we considered in a previous Perspective.
One of the best studied examples of RNA viral persistence of measles virus. After infection the virus can enter the central nervous system (CNS). In time, which can be as long as years after infection, the virus can cause subacute sclerosing panencephalitis(SSPE), a uniformly fatal neurodegenerative disease, although replicative virus cannot generally be recovered from the CNS. However, viral nucleic acids, proteins and nucleocapsids can still be detected in SSPE tissue. These viral footprints appear to represent persistent defective viruses. Analyses of the viral RNA from SSPE shows a variety of mechanisms for the defective persistent nature of the virus, including deletions, mutations (especially of the matrix and fusion protein genes), and evidence of hypermutation. A variety of mutations in the M and F proteins lead to defects in viral budding. Hypermutation is evidenced by massive changes of U to C residues, likely mediated by host-encoded double-stranded RNA-dependent adenosine deaminase. Deletion of the M protein or the F protein tail may allow transmission by cell fusion but preclude cell to cell transmission. It is unclear whether the virus persists in tissues other than the CNS in SSPE.
Another virus in which persistence has been relatively well studied is respiratory syncytial virus (RSV). The presence of DVGs in nasal secretions is associated with various clinical outcomes. Specifically, in children, the presence of DVGs is correlated with more severe disease, higher viral loads, and a greater inflammatory response. In experimentally infected adults, however, the presence of DVGs early after infection were associated with milder disease and low viral loads, whereas the opposite was true when DVGs were present late after infection, indicating the role of DVGs is likely quite complex.
CONCLUSION
The presence of DVGs during infection by RNA viruses appears to almost be the rule rather than the exception, with examples including Ebola, Zika, Chikungunya, and Dengue. For a comprehensive review see ref . DVGs appear to play a variety of conflicting roles. These can include pathogenic and pro-inflammatory roles, but they also can interfere with viral replication and/or stimulate immunity. Whether they exist in long COVID or are generated during the course of infection with SARS-CoV-2 is not clear. If they indeed exist in COVID, they likely represent a two-edged sword that could include a role in long COVID or immune activity.
References
- Radke, J.R. and J.L. Cook, Human adenovirus infections: update and consideration of mechanisms of viral persistence. Curr Opin Infect Dis, 2018. 31(3): p. 251-256.
- Pathak, K.B. and P.D. Nagy, Defective Interfering RNAs: Foes of Viruses and Friends of Virologists. Viruses, 2009. 1(3): p. 895-919.
- Griffin, D.E., Measles virus persistence and its consequences. Curr Opin Virol, 2020. 41: p. 46-51.
- McQuaid, S., et al., Measles virus infection of cells in perivascular infiltrates in the brain in subacute sclerosing panencephalitis: confirmation by non-radioactive in situ hybridization, immunocytochemistry and electron microscopy. Acta Neuropathol, 1993. 85(2): p. 154-8.
- Rima, B.K. and W.P. Duprex, Molecular mechanisms of measles virus persistence. Virus Res, 2005. 111(2): p. 132-47.
- Sidhu, M.S., et al., Defective measles virus in human subacute sclerosing panencephalitis brain. Virology, 1994. 202(2): p. 631-41.
- Cattaneo, R., et al., Biased hypermutation and other genetic changes in defective measles viruses in human brain infections. Cell, 1988. 55(2): p. 255-65.
- Felt, S.A., et al., Detection of respiratory syncytial virus defective genomes in nasal secretions is associated with distinct clinical outcomes. Nat Microbiol, 2021. 6(5): p. 672-681.
- Manzoni, T.B. and C.B. Lopez, Defective (interfering) viral genomes re-explored: impact on antiviral immunity and virus persistence. Future Virol, 2018. 13(7): p. 493-503.