Dengue virus: Urgency in our warming world
Why is this important? In the summer of 2023, Mexico and countries in Central America and the Caribbean were in the news because of extensive outbreaks of the mosquito-borne dengue virus (DENV) (1). These outbreaks were at least partially weather-related: Warmer temperatures exacerbated by El Niño and global warming are linked to heavier-than-normal precipitation in the region, which has facilitated increased reproduction of Aedes aegypti and Aedes albopictus mosquitoes that spread DENV. Outbreaks have been particularly deadly in Peru, Brazil, and Bolivia, but other areas are experiencing major outbreaks, including Bangladesh (2),Chad, which reported its first outbreak (3), and Europe. The city of Paris, France fumigated to kill the spread of these mosquito vectors in 2023 (4).
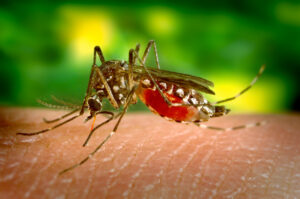
A female Aedes aegypti mosquito takes a blood meal. The mosquito is the primary vector for the spread of Dengue fever. Credit: CDC / James Gathany
Why is DENV different? DENV infections are pernicious for several reasons. For one, a person’s first infection is typically not serious and may even be asymptomatic. Those with symptoms, which are often non-specific and flu-like, may be suspected as having a different viral infection or even malaria. More severe DENV infections occur when a previously infected person is reinfected with a different serotype of the virus. These serotypes are akin to distinct fingerprints, necessitating the immune system to tackle each one separately to elicit responses to subsequent infection that are more nuanced. Only reinfection with the same serotype is durably protected by immunity; reinfection with a different serotype may cause more severe disease, termed “dengue shock syndrome” or “dengue hemorrhagic fever,” depending upon signs in the patient. In total, DENV encompasses four distinguishable serotypes that are based upon their antigenic properties. Variation within serotypes can be substantial (5), and so, while rare, same-serotype reinfections may also be serious.
How exactly is a patient with a second infection of DENV at higher risk of serious disease? After an initial infection, a person’s immune system targets the viral envelope protein with neutralizing antibodies, just as with other enveloped viruses. The initial high concentration (or titers) of these IgG antibodies can be effective against the same DENV serotype – though may offer limited cross-protection against other serotypes. But effectiveness wanes over time as titers fall, particularly in cross-protection against a different serotype. The neutralizing antibodies are still effective against the initial infection serotype, binding and blocking it. They can also protect against viruses within the same serotype, i.e., a homologous serotype. These antibodies can also still bind to the DENV envelope proteins of other serotypes (heterologous serotype), but they cannot block viral entry due to a lack of neutralizing activity. So the lack of neutralizing activity confers an ability to DENV to infect some cells even more efficiently, as cells bear receptors to the antibodies (Fc-gamma receptors ), increasing entry of virus into cells and overall viral loads and thus, the severity disease.
This expansion of the DENV cellular host range and infectious burden is called antibody-dependent enhancement (ADE) or immune enhancement. Interestingly, IgA antibodies induced by infection have been reported to oppose ADE by IgG-enhancing antibodies and can contribute to virus neutralization (6). T-cell responses during secondary infections may also contribute to immune enhancement.
What other viruses are related to DENV? DENV are classified as flaviviruses, a genus that includes yellow fever, Japanese encephalitis, West Nile, and Zika viruses (all spread by mosquitoes), as well as tick-borne viruses like Kyasanur Forest disease, Alkhurma disease, and Omsk hemorrhagic fever (7). DENV has a single positive-stranded RNA genome of about 11,000 nucleotides that encodes both structural and non-structural proteins. Among the non-structural proteins are several that inhibit the type 1 interferon response, the innate immune system’s first line of defense.
Treatments and Vaccines.Currently, there are no available antiviral drugs to treat DENV. In recent years, two vaccines, Qdenga® and Dengavaxia®, have been marketed.
Qdenga is a live-attenuated tetravalent vaccine reported to be >80% effective against hospitalization and 60% effective against disease. However, it is only approved in Europe, the United Kingdom, Brazil, Argentina, Indonesia, and Thailand. Its manufacturer, Takeda, is not pursuing approval in the United States (8).
Dengvaxia®, produced by Sanofi-Pasteur, is a recombinant vaccine with the backbone of a live-attenuated yellow fever virus containing envelope genes from all four dengue serotypes. While efficacy is relatively high in the first 2 years after vaccination, at later times an elevated incidence of hospitalization and severe disease was noted in some persons who were non-DENV-immune upon vaccination (9). The FDA has approved its use only for individuals aged 6-16 living in endemic areas.
New DENV vaccines developed at the U.S. National Institutes of Health and elsewhere have highly promising immunogenicity characteristics, and several are now in clinical trials (9-12). The NIH-developed vaccine induces impressive cross-reacting protection in animals and in Phase 1 human studies, so safety and efficacy from current human Phase 2/3 trials are awaited with great anticipation. The ultimate goal, of course, is a safe, effective, and affordable vaccine for universal use for reducing DENV disease severity (13). However, vaccine development is slowed by the possibility of ADE, so animal studies are especially extensive and human studies are done with extreme care. Any vaccine for DENV must be able to elicit production of broadly neutralizing antibodies against all four viral subtypes, to avoid risking ADE and more severe disease among vaccinees.
Environmental control. Because DENV infection is difficult to prevent and treat, preventive strategies beyond vaccines are essential in the short-term. Mosquito control efforts depend upon insecticides, mosquito netting, and drainage of small containers and reservoirs of standing water, preferred larval habitats of Aedes mosquitoes. There are innovative strategies such as the introduction of mosquito fish (Gambusia sp.) that prey on mosquito larvae. These approaches are only successful with a high degree of community mobilization since they depend on widespread cooperation of the citizenry. In some ecological niches, introduction of mosquito fish has been unsuccessful because they have fed on other mosquito predators.
Other innovative approaches include the use of biologically modified mosquitos, and infecting mosquito populations with intracellular bacteria, the wMel strain of Wolbachia pipientis (14,15). This bacterium reduces the vectorial capacity of the mosquito such that one study reports 86% effectiveness (16) at reducing hospitalization from DENV infections. Large-population effectiveness is not yet clear, but there are no known environmental risks (17). Several other research teams have shown promising results with genetically modified mosquitoes (18), but concerns are substantial as to potential complications of releasing genetically modified organisms into the wild.
The future. The 2024 global situation suggests dengue to still be a growing and serious health menace, exacerbated by mosquito proliferation in temperate regions as the climate warms. Dengue outbreaks are becoming more serious due to climate change and different viral strains are emerging, emphasizing the importance of vaccines and environmental control efforts. Better prevention/mitigation and treatment approaches are needed in nations with warming climates that were not previously dengue endemic. The southern United States and southern Europe are two of many examples. Dengue is a “neglected tropical disease,” not receiving as much attention or investment because it disproportionately affects the world’s poor. Developments of vaccines, antivirals, and biological vector control will make a difference only if accompanied by global investment and advocacy. Vaccine hesitancy (19) will require multidisciplinary research, action, and community engagement.
References
- Dengue – the Region of the Americas, WHO, accessed 9 January 2024 https://www.who.int/emergencies/disease-outbreak-news/item/2023-DON475
- Bangladesh’s worst ever dengue outbreak a ‘canary in the coal mine’ for climate crisis, WHO expert warns, Regan H, CNN, 7 September 2023 https://www.cnn.com/2023/09/07/asia/who-bangladesh-worst-dengue-outbreak-climate-scn-intl-hnk/index.html
- Infectious diseases surveillance update, Islam T, The Lancet Infectious Diseases, 23(12):E518 December 2023. https://doi.org/10.1016/S1473-3099(23)00703-X
- Paris fumigates for tiger mosquitoes as tropical pest spreads across Europe, France 24, 31 August 2023 https://www.france24.com/en/live-news/20230831-paris-fumigates-for-tiger-mosquitoes-as-pest-spreads-in-europe
- Dengue viruses cluster antigenically but not as discrete serotypes. Katzelnick LC et al. Science, 2015, 349(6254):1338-1343. https://doi.org/10.1126/science.aac5017
- DENV-specific IgA contributes protective and non-pathologic function during antibody-dependent enhancement of DENV infection, Wegman, A. D. et al., PLOS Pathogens, 28 August 2023. https://doi.org/10.1371/journal.ppat.1011616
- Flaviviridae, Centers for Disease Control and Prevention (accessed 9 January 2024). https://www.cdc.gov/vhf/virus-families/flaviviridae.html
- Takeda withdraws US application for dengue vaccine candidate, Associated Press, 11 July 2023. https://www.reuters.com/business/healthcare-pharmaceuticals/takeda-withdraws-us-application-dengue-vaccine-candidate-2023-07-11/
- Is new dengue vaccine efficacy data a relief or cause for concern? Thomas SJ, NPJ Vaccines, 15 April 2023. https://www.nature.com/articles/s41541-023-00658-2
- Phase 1 trial to model primary, secondary, and tertiary dengue using a monovalent vaccine, Odio CD, Lowman KE, Law M, et al., BMC Infectious Diseases 2023, 23(1):345. https://doi.org/10.1186/s12879-023-08299-5
- Safety and durable immunogenicity of the TV005 tetravalent dengue vaccine, across serotypes and age groups, in dengue-endemic Bangladesh: a randomised, controlled trial. Walsh MR, Alam MS, Pierce KK, et al., The Lancet Infectious Diseases 2023:S1473-3099(23)00520-0. https://doi.org/10.1016/S1473-3099(23)00520-0
- A Prototype-Pathogen Approach for the Development of Flavivirus Countermeasures, Kuhn RJ, Barrett ADT, Desilva AM, et al., Journal of Infectious Diseases 2023;228(Suppl 6):S398-S413. https://doi.org/10.1093/infdis/jiad193
- Vaccine development for mosquito-borne viral diseases, Huang Z et al., Frontiers in Immunology 12 May 2023, 14:1161149. https://doi.org/10.3389/fimmu.2023.1161149
- Wolbachia strain wMel induces cytoplasmic incompatibility and blocks dengue transmission in Aedes albopictus, Blagrove MS, Arias-Goeta C, Failloux AB, Sinkins SP, Proceedings of the National Academy of Sciences of the USA, 2011, 109(1):255-260. https://doi.org/10.1073/pnas.1112021108
- Establishment of wMel Wolbachia in Aedes aegypti mosquitoes and reduction of local dengue transmission in Cairns and surrounding locations in northern Queensland, Australia. Ryan PA, Turley AP, Wilson G et al., Gates Open Research 2020, 3:1547. https://doi.org/10.12688/gatesopenres.13061.2
- Efficacy of Wolbachia-Infected Mosquito Deployments for the Control of Dengue, Utarini A et al., The New England Journal of Medicine 2021, 384:2177-2186. https://doi.org/10.1056/NEJMoa2030243
- Risk Associated with the Release of Wolbachia-Infected Aedes aegypti Mosquitoes into the Environment in an Effort to Control Dengue, Murray JV, Jansen CC, De Barro P, Frontiers in Public Health, 22 March 2016. https://doi.org/10.3389/fpubh.2016.00043
- Genetically engineered mosquitoes resist spreading any form of dengue, Servick K, Science, 16 January 2020. https://www.science.org/content/article/genetically-engineered-mosquitoes-resist-spreading-any-form-dengue
- 'I was terrified for my child': understanding the link between the Dengvaxia® controversy and the measles vaccine hesitancy in Pasay City, Philippines, Miras AP, Regencia ZJG, Baja ES, Journal of Public Health 2023, 45(4):912-918. https://doi.org/10.1093/pubmed/fdad091