Ebola in Uganda
A moderate sized Ebola outbreak (142 confirmed cases) in Uganda has recently been in the news. Fifty-five people died; the others recovered. It has now largely been controlled, but there are some issues associated with the outbreak that are worth considering. These relate to conventional control by contact tracing and isolation (which was how it was brought under control) and the current vaccine situation (an effective vaccine was not available).
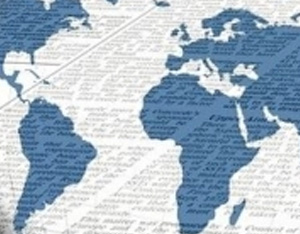
The outbreak in Uganda peaked at 43 cases but declined rapidly thereafter. There was a total of 142 cases with a fatality rate of 39%. Isolation procedures included overnight curfew, closure of places of worship and entertainment, and restrictions on movements in and out of affected districts. Although the efforts ultimately succeeded, there was considerable cost to healthcare workers, with 19 cases and 7 fatalities.
Vaccination was not a factor in these efforts, because the licensed vaccines are not effective against the virus circulating in Uganda. This is because there are five disparate and distinct species or variants; Zaire, Sudan, Tai Forest, Bundibugyo and Reston, the last of which does not appear to cause disease in humans(1). Vaccines that are effective against the Zaire variant exist, but the Sudan variant, which is the one responsible for the Uganda outbreak, is sufficiently different from the Zaire variant that the vaccine is not effective. Indeed, genetic differences among the five variants are substantial. These are largely concentrated within intergenic regions and regions on the surface glycoprotein (GP), nucleoprotein (NP), and polymerase (L) genes (1).
Antibodies in Sudan-infected convalescent sera target four viral proteins, NP, VP30, VP40, and GP (2, 3), and this is also the case for asymptomatic exposed people(2). Interestingly, the strongest activity was against VP40, which is involved in viral assembly and budding. This suggests that a vaccine against VP40 could be useful, especially as it is more genetically stable than GP. Vaccine efforts, however, have focused mainly on the GP, as it is the only protein on the outer virion surface. However, masking of GP epitopes by N- and O-linked glycosylation presents some difficulties for antibody neutralization(4).
The GP is expressed in a variety of different forms(5). The full- length GP, generated by a +1 translational frameshift, is cleaved into subunits S1 and S2, is present on the virion surface as a typical trimer and mediates cell attachment and entry. Full-length GP is also shed by cleavage from its transmembrane anchor and serves both as a neutralizing antibody decoy and an activator of inflammatory cytokine expression from uninfected dendritic cells and macrophages. The N-terminal portion of GP is translated without a frameshift as soluble GP (sGP), which may have immunomodulatory properties and is the most abundant form. A fourth form, small soluble GP (ssGP), is generated by a +2 translational frameshift. It, like sGP, contains the N-terminal region of GP, but is not abundant and its functions, if any, are not well understood.
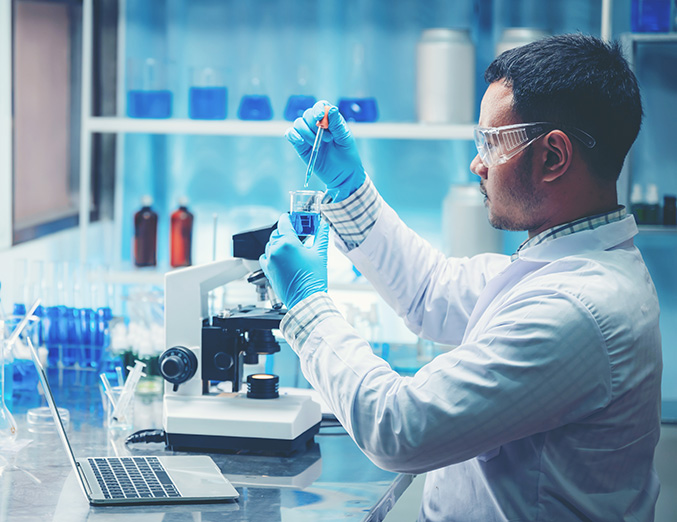
Ebola vaccine development presents some practical difficulties. As outbreaks wax and wane, so does the sense of urgency for vaccine development. Potential test populations tend to be relatively small compared with, for example, Covid, as outbreaks are generally localized. Clearly, challenge trials are not an option because of the deadly nature of Ebola. Thus, the realistic options include human trials for safety and immunogenicity and animal testing for protection. There are two approved vaccines, but both are limited in utility to the Zaire variant. One vaccine, rVSV-ZEBOV (distributed by Merck), uses a replication competent recombinant vesicular stomatitis virus as a vector to deliver the Zaire Ebola GP. It was approved by the US and the European Union and has shown 97% protection from disease in ring vaccinations. The other vaccine, Ad26.ZEBOV and MVA-BN-Filo (approved by the European Union and provided by Johnson and Johnson), is administered in two doses spaced eight weeks apart, making it somewhat less useful in an ongoing breakout, and the real-world efficacy is not yet clear. The early component uses an adenovirus 26 vector to deliver a GP payload, while the later uses a weakened vaccinia virus as a vector.
Other vaccines have been in development but have not yet been approved. One of the issues obviously is variant variability, resulting in a relatively narrow efficacy for a specific vaccine. Given the recent success of mRNA vaccines against Covid and the relative ease with which they can be produced, it is tempting to imagine a multivalent Ebola vaccine containing mRNA for all the variant Ebola GPs, and perhaps including mRNA for VP40. Relevant to this, a recent report showed several GP mRNA vaccines to be 100% protective in a guinea pig animal model(6). This seems a promising avenue for further development. Better approaches are needed.
1. Jun, S.R., et al., Ebolavirus comparative genomics. FEMS Microbiol Rev, 2015. 39(5): p. 764-78.
2. Becquart, P., et al., Identification of continuous human B-cell epitopes in the VP35, VP40, nucleoprotein and glycoprotein of Ebola virus. PLoS One, 2014. 9(6): p. e96360.
3. Sobarzo, A., et al., Profile and persistence of the virus-specific neutralizing humoral immune response in human survivors of Sudan ebolavirus (Gulu). J Infect Dis, 2013. 208(2): p. 299-309.
4. de La Vega, M.A., et al., The multiple roles of sGP in Ebola pathogenesis. Viral Immunol, 2015. 28(1): p. 3-9.
5. Ning, Y.J., et al., The roles of ebolavirus glycoproteins in viral pathogenesis. Virol Sin, 2017. 32(1): p. 3-15.
6. Meyer, M., et al., Modified mRNA-Based Vaccines Elicit Robust Immune Responses and Protect Guinea Pigs From Ebola Virus Disease. J Infect Dis, 2018. 217(3): p. 451-455.